Taking a closer look at damaged DNA
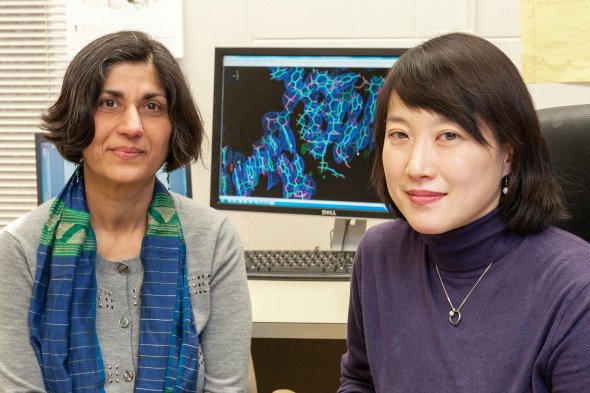
Jung-Hyun Min, assistant professor of chemistry, and Anjum Ansari, professor of physics, study DNA-binding enzymes. Photo: Roberta Dupuis-Devlin
Our entire genetic code is made up of 6 billion base pairs, yet dozens of different enzymes can recognize and interact with very specific patterns, whether they are responsible for turning on — or off — a specific gene, or finding and repairing one type of damage to the DNA itself.
Many, if not most, of these enzymes have a binding site that fits into the site it needs like a lock and key. Still, it boggles the mind to imagine how these enzymes hone in on their targets.
UIC researchers Jung-Hyun Min and Anjum Ansari wondered how a repair enzyme could work if it lacked a lock-and-key binding site. In research published recently in Nature Communications, they found that the enzyme quickly sorts through vast stretches of DNA without wasting time on sites it wasn’t designed to fix and quickly finding the sites where it was needed.
Other DNA-binding enzymes may use this strategy as well.
“If the DNA is normal, the interaction that the protein makes with the DNA is not very tight and the protein is able to hop along at some speed,” said Anjum Ansari, professor of physics and co-principal investigator.
“When the protein encounters a damaged DNA, it’s not quite like a normal DNA, it’s a little twisted,” she said. “The protein stumbles at that spot and gets a little stalled, enough to give it a little bit more time at the damaged site. The longer it sits, the higher the probability that it will open the DNA and initiate repair.”
This “stumble” gives the protein time to flip out one strand of the double strands that make up DNA and begin repair of the other, damaged strand, said Jung-Hyun Min, assistant professor of chemistry and co-principal investigator.
The protein, xeroderma pigmentosum or XPC, is unusual because it can repair different types of damage. This makes it important for the repair of DNA damaged by environmental hazards, like the chemicals released by cigarette smoke, pollutants and fuel combustion, Min said.
To see how the protein binds DNA without this kind of specific binding site, the researchers used a chemical trick to bind the protein to a site on undamaged DNA. To their surprise, they found the protein had flipped open the undamaged DNA.
This suggests that if you hold the repair protein in place long enough anywhere on the DNA, it will open even undamaged DNA, Ansari said.
Using an unusual technique called temperature-jump perturbation spectroscopy, which allowed the researchers to observe the interaction of the protein with DNA millisecond by millisecond, they determined that the protein needed seven milliseconds to flip open DNA at a damaged site.
“We think it could take as much 4,000 times as long to open DNA at an undamaged versus damaged site,” Ansari said. The XPC protein moves too quickly to engage undamaged DNA, but it stalls at a damaged site just long enough to bind the DNA and begin repair.
“This has a potential to explain the kind of phenomena that we couldn’t explain before — how the XPC protein is recruited to unexpected places where it has been found, like transcription sites,” Min said. “It may be that all you need is to bring the protein to these novel sites and stall it for a moment.”
Now that they know what to look for, the two researchers think delay-triggered kinetic gating is a common mechanism, with a role in many, or even all, DNA recognition proteins.
Xuejing Chen and Yogambigai Velmurugu, UIC, are co-first authors on the study. Co-authors are Beomseok Park and Yoonjung Shim, UIC; Guanqun Zheng and Chuan He, University of Chicago; Younchang Kim, Argonne National Laboratory; and Lili Liu and Bennett Van Houten, University of Pittsburgh.