Inner ear has a need for speed
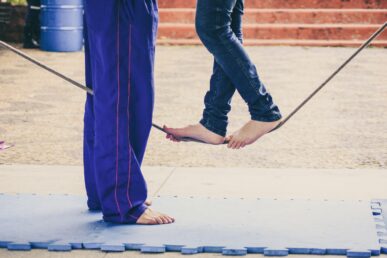
The sensory organs that allow us to walk, dance and turn our heads without dizziness or loss of balance contain specialized synapses that process signals faster than any other in the human body.
In a discovery more than 15 years in the making, a small group of neuroscientists, physicists and engineers from several institutions, including UIC, has unlocked the mechanism of the synapses, paving the way for research that could improve treatments for vertigo and balance disorders that affect as many as 1 in 3 Americans over age 40.
The new study in the Proceedings of the National Academy of Sciences describes the workings of “vestibular hair cell-calyx synapses,” which are found in organs of the innermost ear that sense head position and movements in different directions.
“The vestibular calyx is a wonder of nature,” said Anna Lysakowski, professor of anatomy and cell biology at UIC. “Its large cup-shaped structure is the only one of its kind in the entire nervous system. Structure and function are intimately related, and nature obviously devoted a great deal of energy to produce this structure. We’ve been trying to figure out its special purpose for a long time.”
“Nobody fully understood how this synapse can be so fast, but we have shed light on the mystery,” said Rob Raphael, associate professor of bioengineering in Rice’s George R. Brown School of Engineering.
Synapses are biological junctions where neurons can relay information to one another and other parts of the body. The human body contains hundreds of trillions of synapses, and almost all of them share information via quantal transmission, a form of chemical signaling via neurotransmitters that requires at least 0.5 milliseconds to send information across a synapse.
Prior experiments had shown a faster, “nonquantal” form of transmission occurs in vestibular hair cell-calyx synapses, the points where motion-sensing vestibular hair cells meet afferent neurons that connect directly to the brain. The new research explains how these synapses operate so quickly.
In each, a signal-receiving neuron surrounds the end of its partner hair cell with a large cuplike structure called a calyx. The calyx and hair cell remain separated by a tiny gap, or cleft, measuring just a few billionths of a meter.
From the ion channels expressed in hair cells and their associated calyces, the authors created the first computational model capable of quantitatively describing the nonquantal transmission of signals across this nanoscale gap. Simulating nonquantal transmission allowed the team to investigate what happens throughout the synaptic cleft, which is more extensive in vestibular synapses than other synapses.
“The mechanism turns out to be quite subtle, with dynamic interactions giving rise to fast and slow forms of nonquantal transmission,” Raphael said. “To understand all this, we made a biophysical model of the synapse based on its detailed anatomy and physiology.”
The model simulates the voltage response of the calyx to mechanical and electrical stimuli, tracking the flow of potassium ions through low-voltage-activated ion channels from pre-synaptic hair cells to the post-synaptic calyx.
“The key capability was the ability to predict the potassium level and electrical potential at every location within the cleft,” said Ruth Anne Eatock, professor of neurobiology at the University of Chicago. “This allowed the team to illustrate that the size and speed of nonquantal transmission depend on the novel structure of the calyx. The study demonstrates the power of engineering approaches to elucidate fundamental biological mechanisms, one of the important but sometimes overlooked goals of bioengineering research.”
Raphael, Eatock and Lysakowski co-authored the study with current Rice graduate student Aravind Chenrayan Govindaraju and former Rice graduate student Imran Quraishi, now an assistant professor at Yale University.
The research was supported by the National Institutes of Health (DC012347, DC002290), the Hearing Health Foundation and a seed grant from Rice University’s ENRICH program.
Original story by Rice University.
Categories
Topics
balance disorders, College of Medicine, research, synapses, vertigo, vestibular system